ENERGY HARVESTING FROM FLUTTER
Research activity duration: 2013 – now
Geophysical flows are effective alternative energy sources, due to their large availability in nature and the relevant power density spread all over the world. Recent research studies investigated the possibility of exploiting flow-induced vibrations so that the kinetic energy can be converted into electricity by means of electromagnetic or piezoelectric transducers. Indeed, contrary to the common practice of wind/aeronautical engineering systems can be specifically designed to perform steady-state large-amplitude vibrations, depending on the flow-induced excitation mechanism. The classical flutter entails spontaneously self-sustained oscillations with rapidly increasing amplitudes after the critical threshold. However, only a few research studies are available on the post-critical regime of flutter and reliable predictive models for the large-amplitude pattern are still missing.
The research at CRIACIV focuses on the study of classical-flutter-based power generators, aiming to improve both the scientific knowledge of the post-critical-flutter response and the energy-harvesting design of this type of device. Sectional models with an elongated rectangular cross section of 25:1 (width-to-depth ratio , being the dimension facing the flow) are studied through a large-amplitude aeroelastic setup that enable a motion with heaving and pitching components. The rig also allows to modify some of the governing parameters of the flutter problem and in particular the heaving damping ratio (values up to 18% were achieved through permanent-magnet-based dampers), since this can simulate the operation of an energy-conversion apparatus. Moreover, parametric linear analyses are implemented to find optimal configurations in terms of as low as possible instability threshold.
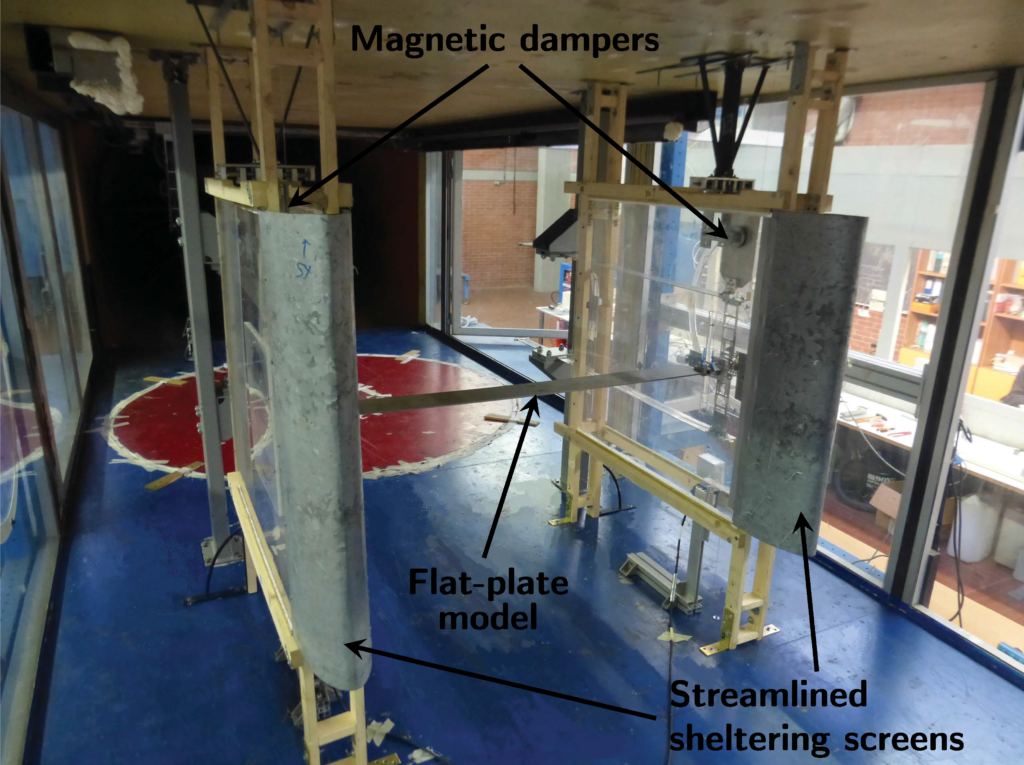
View of the aeroelastic setup, with the eddy-current dampers and the NACA0020 screens used to shelter the rig from the flow.
Several configurations have been investigated, allowing a systematic comparison of the parameters influence. In general, classical-flutter instability manifests with sub-critical bifurcation. Then, limit cycle oscillations are reached at the steady-state regime, showing amplitudes up to about one chord of heaving and 90° of pitching in some cases.
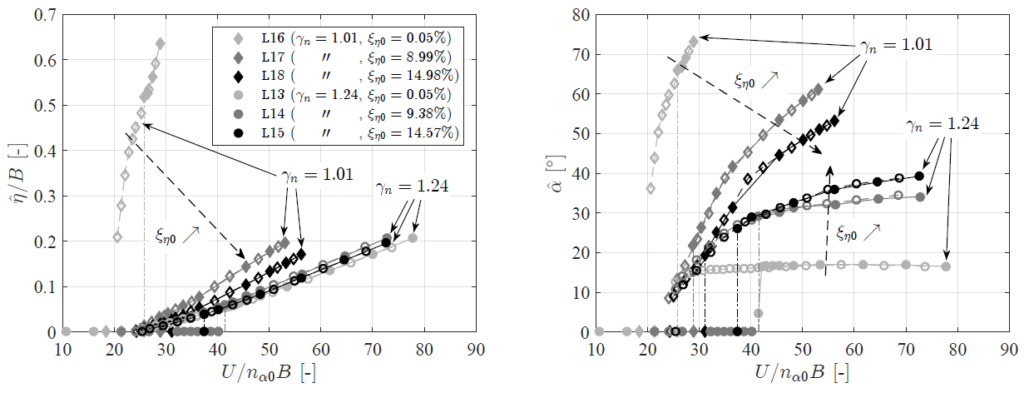
Large-amplitude response of two sets of configurations with different still-air frequency ratio, for different levels of heaving damping. n_α0 is the pitching frequency of oscillation of the uncoupled mechanical system in still air.
Concerning energy-harvesting purposes, a significant reduction of the critical wind speed is achieved by slightly moving the mass centres downstream of the elastic axis. Moreover, the still-air pitching-to-heaving frequency ratio is key parameter that significantly affects the response, and lower values of usually lead to larger heaving amplitudes. The optimal position of the elastic axis Is expected to depend on the heaving-damping level, thus on the amount of energy generation, and it is expected to get closer to the upstream quarter-chord for high damping. Moreover, different behaviours manifest when the heaving damping is increased, depending on the specific set of governing parameters. A peculiar destabilizing effect of the heaving damping is observed for values of around 1.24, for which the critical condition is anticipated and the pitching amplitude increases. By contrast, a general stabilizing effect of damping has been observed for all the other sets of parameters. Finally, flutter-based generators should also be designed with as low as possible inertia with respect to the moved flow, in order to further reduce the critical flow speed.
The sub-critical branch represents an extension of the operative range in which the system can produce energy, if spontaneously reached from higher amplitudes or externally triggered. In particular, during recent investigations of the system in turbulent flow, it has been observed that the buffeting-induced vibrations can in some cases to foster the instability. Furthermore, the system is able to oscillate with large-amplitudes even in a turbulence flow with about 15% of turbulence intensity and integral-length scales of about two times the cross-section width, suggesting the possibility for urban-environment installations.
Next studies will focus on the improvement of the aerodynamic properties of the cross-section and on the investigation of systems with lower inertia.